Significance
Molecular and ion diffusion are fundamental processes with far-reaching implications in various scientific fields, including physics, chemistry, and biology. These processes underlie critical phenomena such as nutrient transport in organisms, pattern formation, chemical reactivity, and even the operation of modern batteries. The study of diffusion has been greatly facilitated by techniques like nuclear magnetic resonance (NMR) spectroscopy, which have been widely used for decades. However, conventional NMR methods have their limitations, particularly when it comes to studying diffusion at the microscale. In a new study led by Dr. Dominik Bucher from the Technical University of Munich and published in the Journal Science Advances, a novel approach to overcoming these limitations was presented. The study leverages the unique properties of nitrogen-vacancy (NV) centers in diamond, which serve as atom-sized quantum sensors for magnetic fields. This innovation allows for the spatially resolved detection of diffusion phenomena at unprecedented length scales, providing valuable insights into complex systems. Conventional NMR techniques, while highly valuable, encounter challenges when applied to microscale diffusion studies. These challenges primarily revolve around limitations related to signal-to-noise ratio (SNR), spatial resolution, and the inherent diffusion weighting introduced by the NMR methodology itself.
The nitrogen-vacancy (NV) center in diamond offers an elegant solution to these challenges. This atom-sized quantum sensor is capable of optically detected magnetic resonance (ODMR) experiments, allowing the translation of local magnetic fields into optical signals. The NV center’s unique properties have enabled researchers to conduct NMR experiments at unprecedented length scales, with high spatial resolution and sensitivity.
NV-NMR spectroscopy represents a powerful tool for investigating diffusion at the microscale due to several key advantages such as optical readout where the NV center’s fluorescence properties enable spatially resolved NMR measurements, providing precise localization of diffusion phenomena. Secondly, high spatial resolution, where the detection volume in NV-NMR corresponds to the laser spot size and the thickness of the NV layer. This enables the local detection of NMR signals on a length scale comparable to or smaller than the average distance a water molecule diffuses within the timescale of a typical NMR experiment.
The authors’ experimental setup for NV-NMR spectroscopy involves two critical components: diffusion encoding using magnetic field gradient pulses during a spin-echo sequence and the detection of the corresponding NMR signal with an NV ensemble. They designed and fabricated magnetic field gradient coils to meet the specific requirements of NV-NMR spectroscopy. These coils were capable of producing gradient strengths on par with top-performance whole-body clinical MRI scanners, enabling the precise control of diffusion encoding. The pulse sequence used in NV-NMR spectroscopy, known as pulsed gradient spin echo (PGSE), allows for the detection of diffusion within picoliter sample volumes. PGSE experiments were conducted to measure water flow within a microfluidic channel and assess the influence of a water-soluble polymer on water diffusivity. Additionally, the technique demonstrated its capability to detect local water diffusion within a microstructure, showing anisotropic diffusion based on the local geometry and structure. The authors successfully measured the flow velocity of water within a microfluidic channel using PGSE NV-NMR spectroscopy. This allowed for the precise determination of flow rates, and the results were in good agreement with theoretical predictions. They employed NV-NMR spectroscopy to measure the diffusion coefficient of water in a microfluidic channel. By introducing a water-soluble polymer, the influence on water diffusivity was examined. The results showed excellent agreement with theoretical simulations. The study also investigated the time dependence of the apparent diffusion coefficient (ADC) within a microfluidic channel. It revealed that as the free diffusion time increased, more molecules interacted with confinement boundaries, leading to a decrease in ADC. This phenomenon was both experimentally observed and numerically simulated. Furthermore, the researchers designed a microfluidic structure with different channel sizes and orientations to probe water diffusion spatially. The diffusion tensor was calculated for various locations and directions within the structure. The results demonstrated changes in ADC based on the channel dimensions and were consistent with simulations.
The new NV-NMR spectroscopy technique represents a significant advancement in the study of molecular diffusion at the microscale. While the current spatial resolution is on par with conventional diffusion MRI, the technique holds the potential for even higher spatial resolution by further reducing the thickness of the NV center-doped layer and laser spot size. This advancement could enable the investigation of diffusion properties at the single-cell level, offering valuable insights into biological systems. Moreover, the ability to apply very strong magnetic field gradients, facilitated by the small length scale of NV-NMR, opens up new possibilities for studying various phenomena, including slowly diffusing spins and ion diffusion in solid-state materials. Technical developments may further enhance the technique’s capabilities, making it a versatile tool for diverse research applications. In summary, the study led by Dr. Dominik Bucher and his team demonstrates the transformative potential of NV-NMR spectroscopy in advancing our understanding of molecular diffusion. This innovative approach has the potential to reshape how we study and characterize diffusion processes in a wide range of scientific disciplines, from biology to materials science and beyond. As the technique continues to evolve, it holds promise for unlocking new discoveries and insights at the microscale, with implications for both fundamental research and practical applications.
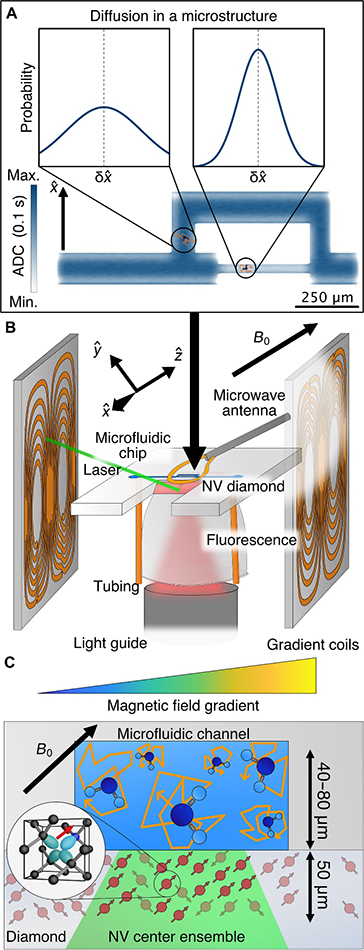
Reference
Bruckmaier F, Allert RD, Neuling NR, Amrein P, Littin S, Briegel KD, Schätzle P, Knittel P, Zaitsev M, Bucher DB. Imaging local diffusion in microstructures using NV-based pulsed field gradient NMR. Sci Adv. 2023 ;9(33):eadh3484. doi: 10.1126/sciadv.adh3484.