Significance
Vapor-liquid equilibrium (VLE) refers to a condition where a liquid and its vapor (gas phase) coexist at a specific temperature and pressure in a state of dynamic equilibrium. In this state, the rate at which molecules evaporate from the liquid phase to the vapor phase is equal to the rate at which they condense back into the liquid, resulting in no net change in the composition or amount of the two phases over time. The VLE concept is fundamental in chemical engineering and thermodynamics for the design and analysis of separation processes such as distillation, absorption, and extraction. Understanding VLE is crucial for determining the optimal conditions under which components of a mixture can be efficiently separated based on their volatilities. To study the VLE of binary mixtures involving ammonia and supercritical gases such as argon, methane, hydrogen, nitrogen, and oxygen, a new study published in Journal of Chemical & Engineering Data and conducted by graduate student Erich Mace and Professor Jadran Vrabec from the Technical University of Berlin in Germany, the authors emphasized the importance of understanding the thermophysical properties of ammonia and its mixtures for various industrial applications. The research utilizes advanced computational methods, specifically Monte Carlo and molecular dynamics simulations, to generate over 2000 data points across different temperatures and pressures, offering insights into the phase behaviors of these mixtures.
The authors investigated mixtures like argon–ammonia, methane–ammonia, hydrogen–ammonia, nitrogen–ammonia, and oxygen–ammonia and the findings significantly extended the database of ammonia mixtures, providing new, highly accurate thermophysical data. Indeed, ammonia’s role in various industrial applications—from refrigeration systems to power generation and emissions control technologies necessitates a deep understanding of its thermophysical properties in mixtures. The authors’ work significantly enriches the database of ammonia mixtures, particularly at high pressures, facilitating better process design and innovation in utilizing ammonia. Moreover, the authors employed Monte Carlo and molecular dynamics simulations to predict the VLE behavior of the binary mixtures, and demonstrated the power of computational methods in reducing the need for experimental work, especially under hazardous or extreme conditions. The estimated uncertainties in the generated data are regularly less than 5%, showcasing the reliability of the computational approaches used. This accuracy is important for the design and optimization of chemical processes involving these mixtures. Furthermore, the researchers provided detailed information on Henry’s constants and high-pressure fluid-phase equilibria, important for process design and simulation in chemical engineering and related fields.
Given the global push for sustainable and green technologies, ammonia’s potential as a renewable energy carrier and in carbon capture applications positions this study as a pivotal reference point for future research aimed at environmental sustainability. The detailed data provided on ammonia mixtures can help optimize processes for cleaner energy production and more efficient carbon management strategies. The study’s insights into the thermophysical properties of ammonia mixtures underpin the development of more efficient refrigeration systems and power generation cycles. Such applications are critical in industries ranging from food preservation to thermal power plants, where efficiency gains can lead to significant energy savings and reduced environmental impact. By understanding the behavior of ammonia mixtures, particularly in applications like selective catalytic reduction for controlling nitrogen oxides emissions, the study contributes to the design of more effective systems for reducing air pollution from industrial sources.
In conclusion, the study conducted by Erich Mace and Prof. Jadran Vrabec on the high-pressure fluid-phase equilibria and Henry’s constants of supercritical gases in ammonia carries significant scientific and industrial implications. By leveraging Monte Carlo and molecular dynamics simulations, the study represents a significant advancement in the computational investigation of chemical processes. It increase our fundamental understanding of fluid-phase behavior at high pressures and supports the advancement of technologies that utilize ammonia and supercritical gases, highlighting the ongoing relevance of ammonia in modern industrial and environmental applications. This approach not only reduces reliance on labor-intensive and potentially hazardous experimental procedures but also opens new avenues for exploring complex systems under a wide range of conditions that might be difficult or impossible to replicate experimentally.
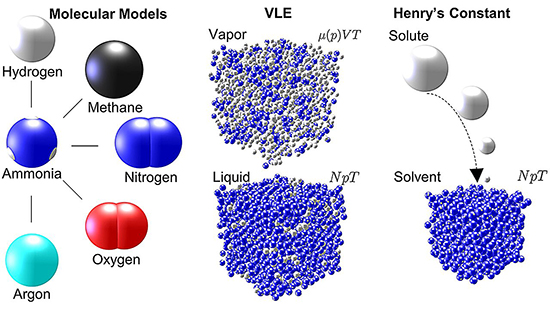
Reference
Erich J. Mace and Jadran Vrabec*. High-Pressure Fluid-Phase Equilibria and Henry’s Constants of Supercritical Gases in Ammonia. J. Chem. Eng. Data 2024, 69, 2, 573–589